IEEE 1679.1-2017 pdf download.IEEE Guide for the Characterization and Evaluation of Lithium-Based Batteries in Stationary Applications
5. Technology description
5.1 General
Refer to Clause 5 of IEEE Std 1679-2010. This clause describes the main lithium-based technologies that are either used or are being considered for stationary battery applications.
These include lithium-ion (Li- ion), lithium-ion polymer (LiPo), lithium-metal polymer (LMP) and lithium-sulfur (Li-S). Lithium-based batteries use lithium metal or some other source of lithium ions in the negative electrode. During the battery discharge, the lithium ions travel to the positive electrode, which can be one of various materials, including a transition metal oxide, a transition metal phosphate, a sulfur compound, or even oxygen in the atmosphere or water. The electrolyte is typically a conductive salt in an organic liquid solution, or a conductive polymer.
The information provided in this clause relates predominantly to Li-ion technology, as this technology is by far the most widely used. Differences between Li-ion and other lithium battery technologies are highlighted where appropriate. Lithium-based batteries typically comprise cells and associated management systems. In many cases these batteries are assembled by the cell manufacturer, and in others an integrator assembles cells from a third party with the integrator’s management systems. In both cases, the provider is referred to in this document as the manufacturer.
5.2 Storage medium
5.2.1 General Li-ion batteries are the most common lithium-based battery type, and include a wide range of chemistries that all operate in the same general manner. The traditional Li-ion battery has a negative electrode (commonly referred to as the anode), typically a layered carbon; a positive electrode (cathode), typically a lithiated metal oxide or lithiated metal phosphate; and an electrolyte containing a lithium salt in an organic solvent. On discharge, lithium ions flow from between the carbon layers in the negative to the oxide layers in the positive. On charge, the lithium ions flow in reverse, moving back into the carbon layers. This process is known as intercalation and referred to colloquially as a ‘rocking chair’ reaction.
Figure 1 shows a representation of the Li-ion reaction mechanism for a typical chemistry with metal oxide positive and carbon (graphite) negative materials.
5.2.2 Active materials
The following lists show common commercial materials used in Li-ion cells. Some roducts use blends of two or more materials. Electrode materials are often referred to by abbreviations, hich are provided in parentheses where applicable.
Negative (anode)
Unstructured/hard carbon
Layered carbon (graphite)
Lithium titanate (LTO)
Silicon compounds
Positive (cathode)
Lithium cobalt oxide (LCO)
Lithium manganese oxide (LMO)
Lithiated mixed-metal oxide [e.g., lithium nickel-manganese-cobalt oxide (NMC), lithium nickel-
cobalt-aluminum oxide (NCA)]
Lithium iron phosphate (LFP)
Electrolyte
Lithium hexafluorophosphate (LiPF 6 ) salt in organic carbonate solution
Lithium tetrafluoroborate (LiBF 4 ) salt in organic carbonate solution
The electrolyte formulation is proprietary to each manufacturer and is critical to the life of the product, particularly regarding the stability of positive materials with high electrode potentials. The formulation can also affect the cell performance, especially at low temperature, and can help to stabilize the solid- electrolyte interphase (see 5.2.3). The electrolyte may also contain additives with specific functions, for example to produce gas during a thermal runaway event, to assist cell venting (see 6.4.1.4).
5.2.3 Material characteristics
The characteristics of the final product vary markedly depending on the materials used. For example, Figure 2 provides a simple comparison of various positive active materials for a range of attributes. Precise material characteristics vary; consult the manufacturer for information on specific products. Better relative performance is indicated by positions at the outside edge of each chart and worse performance by positions towards the center. The listed attributes are as follows:
Calendar life at 20 °C to 25 °C
Calendar life at high temperature (>40 °C).IEEE 1679.1 pdf download.IEEE 1679.1-2017 pdf download
IEEE 1679.1-2017 pdf download
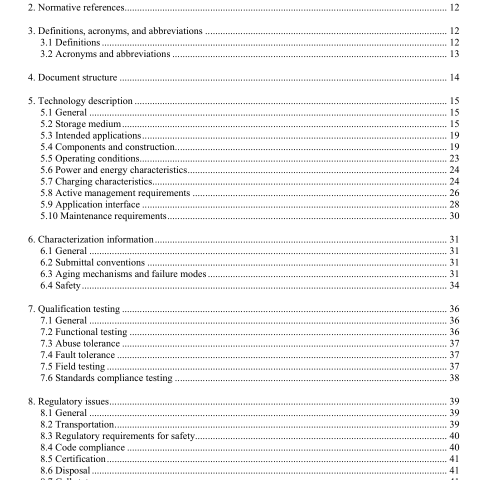
Leave a Reply